Gluconeogenesis: A Comprehensive Overview of its Pathways and Mechanisms
Gluconeogenesis is a complex metabolic pathway that involves a series of enzyme-catalyzed reactions to produce glucose from non-carbohydrate precursors. It is essentially the reverse of glycolysis, with several key steps bypassed to overcome the thermodynamic barriers of converting pyruvate back into glucose. Researchers at the Max Planck Institute of Biochemistry have utilized molecular biology techniques to uncover the key enzymes and signaling pathways involved in the process. These studies have provided valuable insights into the molecular basis of gluconeogenesis.
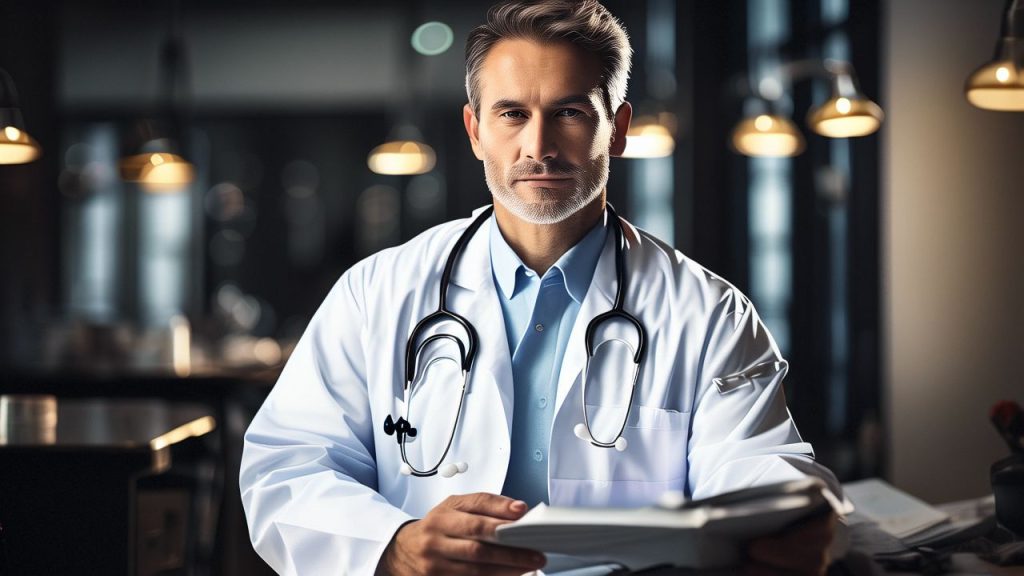
Here is a detailed description of the gluconeogenesis pathway:
- Conversion of Pyruvate to Oxaloacetate:
- Pyruvate, derived from lactate, amino acids, or glycerol, is transported into the mitochondria.
- Pyruvate is carboxylated to oxaloacetate by the enzyme pyruvate carboxylase, using biotin as a cofactor and ATP as an energy source.
- Transport of Oxaloacetate:
- Oxaloacetate is converted to malate by the enzyme malate dehydrogenase, which can be transported out of the mitochondria into the cytosol.
- Conversion of Malate back to Oxaloacetate:
- In the cytosol, malate is regenerated back to oxaloacetate by malate dehydrogenase.
- Oxaloacetate is then phosphorylated to phosphoenolpyruvate (PEP) by the enzyme phosphoenolpyruvate carboxykinase (PEPCK), consuming GTP.
- Conversion of PEP to Fructose-1,6-bisphosphate:
- PEP is converted to fructose-1,6-bisphosphate by the enzyme fructose-1,6-bisphosphatase, which is different from the glycolytic enzyme phosphofructokinase-1.
- Conversion of Fructose-1,6-bisphosphate to Glucose-6-phosphate:
- Fructose-1,6-bisphosphate is hydrolyzed to free glucose-6-phosphate and inorganic phosphate by the enzyme fructose-1,6-bisphosphatase.
- Conversion of Glucose-6-phosphate to Glucose:
- Glucose-6-phosphate is converted to free glucose by the enzyme glucose-6-phosphatase, which is primarily found in the liver and kidneys. This step is critical and is the key regulatory point in gluconeogenesis.
The regulation is tightly controlled to ensure that glucose production occurs only when needed. Key regulatory enzymes include pyruvate carboxylase, phosphoenolpyruvate carboxykinase, fructose-1,6-bisphosphatase, and glucose-6-phosphatase. These enzymes are allosterically and hormonally regulated, with factors such as glucagon, cyclic AMP, insulin, and ATP levels playing important roles in controlling their activity.
Gluconeogenesis is an energy-intensive process, as it requires ATP and GTP for several of the steps. The primary substrates for gluconeogenesis include lactate, glycerol, certain amino acids (both glucogenic and ketogenic), and pyruvate derived from alanine via the alanine cycle.
This pathway is essential during periods of fasting, starvation, low carbohydrate intake, and other conditions where the body needs to maintain blood glucose levels to provide energy to the brain and red blood cells, which depend almost exclusively on glucose as a fuel source.
Significance and functions
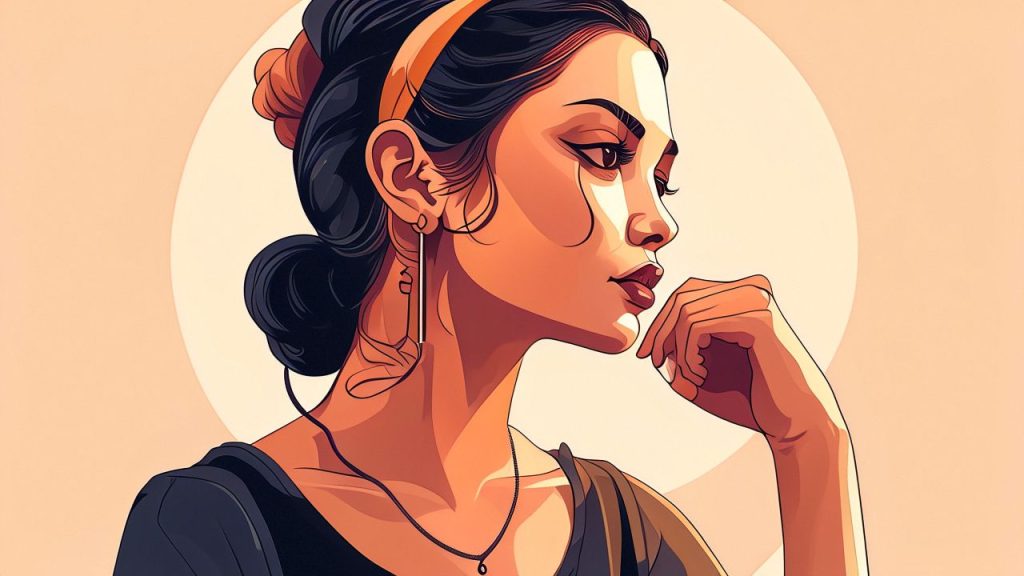
Gluconeogenesis is a vital metabolic pathway that plays a crucial role in maintaining blood glucose levels within a narrow range, ensuring that the body’s energy needs are met, particularly during periods of fasting, starvation, or low carbohydrate intake. The significance and functions of gluconeogenesis can be summarized as follows:
- Energy Homeostasis:
- Gluconeogenesis provides a constant supply of glucose to the bloodstream, which is essential for organs, such as the brain and red blood cells, that rely heavily on glucose as their primary energy source.
- Preventing Hypoglycemia:
- By generating glucose from non-carbohydrate precursors, gluconeogenesis prevents blood sugar levels from dropping too low, which can lead to hypoglycemia and its associated symptoms, including dizziness, confusion, and, in severe cases, coma or death.
- Utilization of Alternative Fuel Sources:
- Gluconeogenesis allows the body to use substrates such as lactate, glycerol, and certain amino acids to produce glucose. This is particularly important during prolonged fasting or intense exercise when glycogen stores are depleted.
- Conservation of Proteins:
- By using amino acids as substrates, the body can spare proteins from being used as an energy source, thus preserving muscle mass and other vital proteins.
- Integration with Other Metabolic Pathways:
- Gluconeogenesis is integrated with other metabolic pathways, such as the tricarboxylic acid cycle (TCA cycle) and fatty acid oxidation, allowing for the interconversion of metabolites and the efficient use of energy resources.
- Regulation of Blood Glucose:
- Gluconeogenesis is hormonally regulated, primarily by glucagon and insulin, which control the activity of key enzymes in the pathway. This regulation helps maintain blood glucose homeostasis.
- Kidney Function:
- In the kidneys, gluconeogenesis contributes to the reabsorption of glucose from the tubular fluid back into the bloodstream, preventing the loss of glucose through urine.
Overall, gluconeogenesis is a critical adaptation that allows organisms to survive periods of glucose scarcity by synthesizing glucose from non-carbohydrate precursors. It is an essential process for maintaining energy balance and ensuring the proper functioning of glucose-dependent tissues and organs.
Relationship between Glycolysis and gluconeogenesis
Glycolysis and gluconeogenesis are two interconnected metabolic pathways that have opposite directions but share several common steps. They are part of the broader glucose metabolism system in the body, and their relationship can be described as follows:
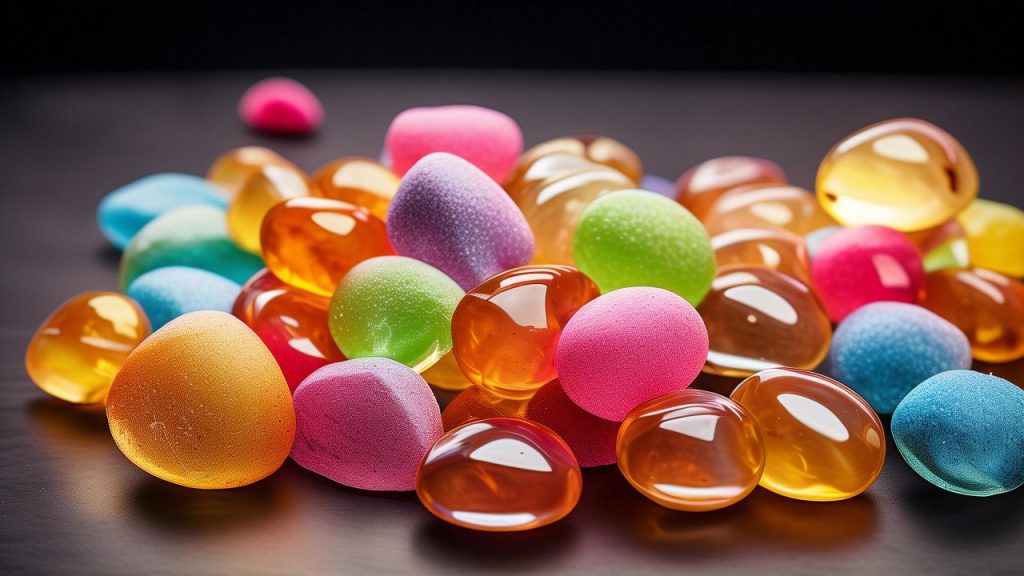
- Opposite Reactions:
- Glycolysis is the catabolic process by which glucose is broken down into pyruvate, generating a small amount of ATP and NADH in the process. It occurs in the cytoplasm of cells and is the first step in the extraction of energy from glucose.
- Gluconeogenesis, on the other hand, is an anabolic process that synthesizes glucose from non-carbohydrate precursors, such as pyruvate, lactate, glycerol, and certain amino acids. It is essentially the reverse of glycolysis and occurs primarily in the liver and, to a lesser extent, in the kidneys.
- Shared Intermediates:
- Both pathways share several intermediates, including glucose-6-phosphate, fructose-1,6-bisphosphate, and phosphoenolpyruvate (PEP). However, because some of the reactions in glycolysis are thermodynamically irreversible under physiological conditions, gluconeogenesis uses a different set of enzymes to bypass these steps.
- Regulatory Distinction:
- The enzymes involved in the shared steps of glycolysis and gluconeogenesis are often different isoforms that are regulated independently. For example, the glycolytic enzyme phosphofructokinase-1 (PFK-1) is allosterically inhibited by high levels of ATP and citrate, while the gluconeogenic enzyme fructose-1,6-bisphosphatase is activated by high levels of ATP and citrate.
- Energy Requirements:
- Glycolysis is a net producer of ATP (although it consumes ATP in the initial steps), while gluconeogenesis is a net consumer of ATP because it must overcome the energy barriers of converting pyruvate back into glucose.
- Physiological Roles:
- Glycolysis is the primary means by which cells produce energy in the form of ATP, especially in situations where oxygen availability is limited, such as during intense exercise.
- Gluconeogenesis is essential for maintaining blood glucose levels during fasting, starvation, or other conditions where glucose availability is low. It ensures a constant supply of glucose to the brain and red blood cells, which depend on glucose as their main energy source.
In summary, glycolysis and gluconeogenesis are interrelated pathways that balance the breakdown and synthesis of glucose in the body. They are regulated by different enzymes and respond to the body’s energy needs and nutrient availability, ensuring that glucose homeostasis is maintained under various physiological conditions.
Changes that may occur in gluconeogenesis in diabetic patients
In individuals with diabetes, the process can undergo several changes, which are primarily influenced by the type and severity of the diabetes, as well as the presence of other metabolic disturbances. Here are some of the key changes that may occur in gluconeogenesis in diabetic patients:
- Increased Gluconeogenesis:
- In diabetes, especially in uncontrolled or insulin-deficient conditions, there is often an increase in gluconeogenesis. This is because the body tries to maintain normal blood glucose levels despite impaired glucose uptake by cells and tissues.
- Altered Enzyme Activity:
- The activity of key gluconeogenic enzymes may be upregulated in diabetes. For example, the expression and activity of phosphoenolpyruvate carboxykinase (PEPCK) and fructose-1,6-bisphosphatase (FBPase), which are rate-limiting enzymes in gluconeogenesis, are often increased.
- Enhanced Substrate Availability:
- Diabetic conditions can lead to increased availability of gluconeogenic substrates such as lactate, glycerol, and amino acids. This is due to enhanced breakdown of proteins and fats, as well as increased glycolysis and lactate production.
- Insulin Resistance:
- In type 2 diabetes, insulin resistance can lead to elevated levels of glucagon, which is a potent stimulator of gluconeogenesis. Glucagon promotes the breakdown of liver glycogen (glycogenolysis) and also directly stimulates gluconeogenic enzyme expression.
- Ketogenesis:
- In some forms of diabetes, particularly in uncontrolled type 1 diabetes, the increased production of ketone bodies can occur due to the excessive availability of acetyl CoA, which can be used as an alternative substrate for gluconeogenesis.
- Hyperglycemia and Ketoacidosis:
- The combination of increased gluconeogenesis, impaired insulin action, and elevated counter-regulatory hormones like glucagon can lead to persistent hyperglycemia and, in severe cases, diabetic ketoacidosis.
- Metabolic Inflexibility:
- Diabetes can lead to a state of metabolic inflexibility, where the body’s ability to switch between using glucose and other fuel sources is impaired. This can result in an over-reliance on gluconeogenesis to maintain blood glucose levels.
These changes in gluconeogenesis contribute to the metabolic abnormalities seen in diabetes and can have significant implications for the management and treatment of the condition. It is important for diabetic patients to maintain good blood glucose control to minimize the impact of these changes on overall health.
Pingback: The concept, source and destination of blood glucose - Disease and Health
Pingback: The concept, source and destination of blood glucose - Disease and Health